Narrowing the gap between physics and chemistry
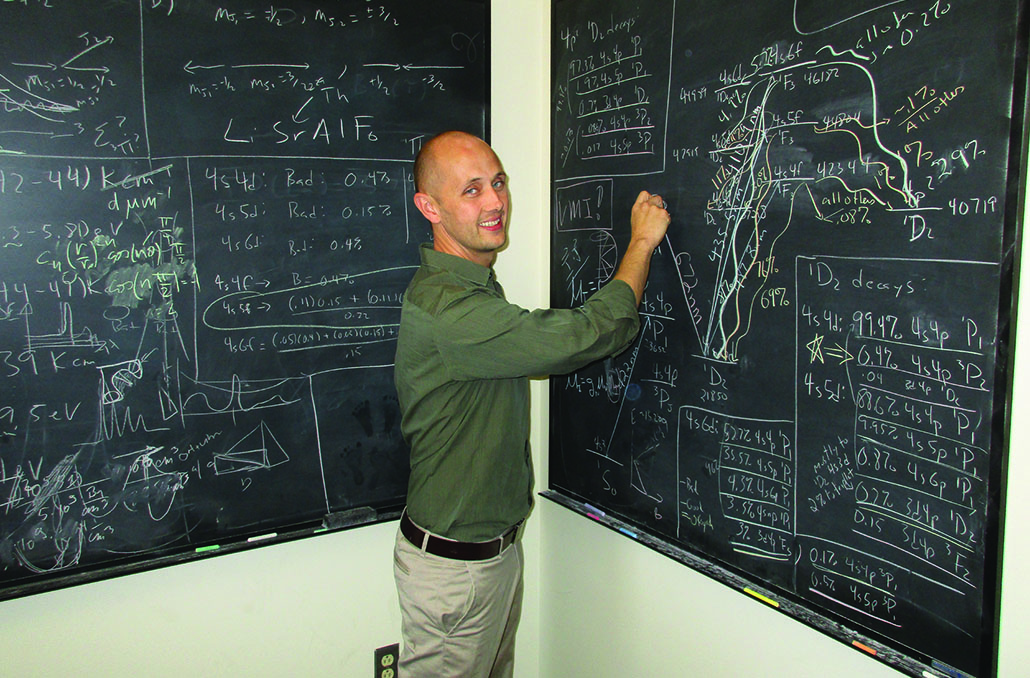
Eric Hudson
By Stuart Wolpert
UCLA physicists have pioneered a method for creating a unique new molecule that could lead to many useful applications in medicine, food science and other fields. Their research, published in the journal Science, also shows how chemical reactions can be studied on a microscopic scale using tools of physics.
For the past 200 years, scientists have developed rules to describe chemical reactions that they have observed, including reactions in food, vitamins, medications and living organisms. One of the most ubiquitous is the “octet rule,” which states that each atom in a molecule that is produced by a chemical reaction will have eight outer orbiting electrons. (Scientists have found rare exceptions to the rule).
The molecule created by professor Eric Hudson and colleagues violates that rule. Barium-oxygen-calcium, or BaOCa+, is the first molecule ever observed by scientists that is composed of an oxygen atom bonded to two different metal atoms.
Normally, one metal atom (either barium or calcium) can react with an oxygen atom to produce a stable molecule. However, when the UCLA scientists added a second metal atom to the mix, a new molecule, BaOCa+, which no longer satisfied the octet rule, had been formed.
Ultra-cold physics tools
Other molecules that violate the octet rule have been observed before, but the UCLA study is among the first to observe such a molecule using tools from physics – namely lasers, ion traps and ultra-cold atom traps.
Hudson’s laboratory used laser light to cool tiny amounts of the reactant atoms and molecules to an extremely low temperature – one one-thousandth of a degree above absolute zero – and then levitate them in a space smaller than the width of a human hair, inside of a vacuum chamber. Under these highly controlled conditions, the scientists could observe properties of the atoms and molecules that are otherwise hidden, and the “physics tools” they used enabled them to hold a sample of atoms and observe chemical reactions one molecule at a time.
The ultra-cold temperatures used in the experiment can also be used to simulate the reaction as it would occur in outer space. That could help scientists understand how certain complex molecules, including some that could be precursors to life, came to exist in space, Hudson said.
The researchers found that when they brought together calcium and barium methoxide inside of their system under normal conditions, they would not react because the atoms could not find a way to rearrange themselves to form a stable molecule. However, when the scientists used a laser to change the distribution of the electrons in the calcium atom, the reaction quickly proceeded, producing a new molecule, CaOBa+.
The Hudson group’s approach is part of a new physics-inspired subfield of chemistry that uses the tools of ultra-cold physics, such as lasers and electromagnetism, to observe and control how and when single-particle reactions occur.
Practical applications
Graduate student Prateek Puri, the project’s lead researcher, said the experiment demonstrates not only how these techniques can be used to create exotic molecules, but also how they can be used to engineer important reactions. The discovery could ultimately be used to create new methods for preserving food and developing safer medications.
“Experiments like these pave the way for developing new methods for controlling chemistry,” Puri said. “We’re essentially creating ‘on buttons’ for reactions.”
Food decays, he said, when undesired chemical reactions occur between food and the environment. Similarly, many medicines induce chemical reactions that can cause harm to the body. Perhaps in the future, scientists could prevent these types of reactions from occurring, or reduce their frequency, Hudson said.
Hudson said one key to the success of the new study was the involvement of experts from various fields: experimental physicists, theoretical physicists and a physical chemist.
Co-authors of the study are Christian Schneider, a UCLA research scientist; Michael Mills, a UCLA graduate student; Ionel Simbotin, a University of Connecticut physics postdoctoral scholar; John Montgomery Jr., a University of Connecticut research professor of physics; Robin Côté, a University of Connecticut professor of physics; and Arthur Suits, a University of Missouri professor of chemistry. The research was funded by the National Science Foundation and Army Research Office.
Findings lead to new areas of study
“We realized we could create molecules in ways we had not appreciated before,” Hudson said. “That led us to start thinking about designing molecules differently.”
As an outgrowth of this insight, a research team involving Hudson and led by Wesley Campbell, associate professor of physics, has been awarded a three-year, $2.7 million U.S. Department of Energy Quantum Information Science Research Award. The emerging, multidisciplinary field of quantum information science is expected to lay the foundation for the next generation of computing and information processing, as well as many other innovative technologies.
Quantum computers, once fully developed, will be capable of solving large, extremely complex problems that are beyond the
capacity of today’s most powerful supercomputers. Among other applications, quantum systems hold the promise of potentially
exquisitely sensitive sensors, with a variety of possible medical, national security and scientific applications.
With this funding, faculty in chemistry and physics will develop and study “molecules functionalized with optical cycling centers,” accelerating research into next-generation chemical systems for quantum information storage and processing.
The primary investigators of this grant are Campbell; Hudson; Justin Caram, a UCLA assistant professor of chemistry; Anastassia Alexandrova, UCLA associate professor of chemistry and biochemistry; Anna Krylov, USC professor of chemistry; John Doyle, Harvard University professor of physics; and Nick Hutzler, Caltech assistant professor of physics.